Why monarchs are an enzyme – Part 2
Tuesday, February 25th, 2020 at 7:47 am by Chip TaylorFiled under Monarch Biology | Comments Off on Why monarchs are an enzyme – Part 2
See Why monarchs are an enzyme – Part 1 posted earlier this month.
What the heck is realized fecundity/fertility and why is it important?
A term I mention from time to time in my talks is realized fecundity. Add to that, I might mention fertility, reproductive success and age to first reproduction. I first encountered these terms when taking an ecology course in graduate school in the late 1960s. There was a unit in the course that dealt with demography – birth and death rates, stable age distributions and factors leading to declines or growth of populations. Among these lessons were a few exercises designed to inform the students of factors that most strongly influenced population growth. The two that stood out were realized fecundity and age to first reproduction. Both are key to understanding population growth in monarchs and inter-annual variations in the sizes of the population at the end of the growing season.
When assessing population growth, the focus is on females. Each monarch female has a potential to produce offspring – a maximum number of eggs that could be laid given the size of the female, the fat body carried over from the larval stage, it’s inherent fitness as defined by its genes, etc. Total lifespan is a factor as well. There are only so many wing beats and degree days per life time. These are all intrinsic factors, that is, properties of each female. Realized fecundity deals with extrinsic factors that have the effect of limiting the number of eggs laid by a female in her lifetime. The list of extrinsic factors is long and involves both physical factors such temperature, precipitation, and wind speed as well as biological factors that include plant quality, nectar availability, number of and fertility of mates, predators, etc. In addition, we need to consider that there may be a cost in terms of eggs not laid due to search time required to find suitable host plants and nectar sources. Search time involves habitat fragmentation which I may get to in other posts.
A basic tenant of population growth is that populations with a short age to first reproduction grow faster than populations in which reproduction is delayed. When talking about monarchs, age to first reproduction can be defined as the interval from when an egg is laid until a female that has developed from that egg lays her first egg. That interval can be as short as 30 days (4 egg, 12 larva, 10 pupa, 4 mating to first egg) and at least as long as 50 days in the spring. This range is due to differences in the temperatures experienced throughout the developmental period. In other words, the temperatures experienced throughout development determine age to first reproduction. As we will see, the distribution of eggs across the latitudes in the spring has a big role in determining age to first reproduction and therefore population growth.
While I used the potential egg laying capacity of individual females to introduce this topic, we need to consider females as a group or cohort to determine the impact of extrinsic factors at the population level. So, what are the conditions that would enable a cohort of females to achieve a high-level egg laying or, conversely to reduce egg laying? I’ve examined the weather conditions during the annual cycle for the eastern monarch population for every year since 1994. That analysis has yielded lists of factors that favor and do not favor monarch population growth (Tables 1 and 2). As you go down the list in each of these figures, you will note that a number of the factors listed, such as winter mortality or survival as monarchs migrate north from the overwintering sites into Texas in March, have to do with losses that ultimately define cohort size. Some unknown portion of that mortality may be due to the condition of the butterflies that arrived at the overwintering sites in the fall. For example, butterflies that experienced drought, either during development as a larva or during the migration itself, are more likely to die during the winter and migration north than butterflies that developed and migrated under more favorable conditions. Further, it is probably the case that the potential reproductive capacity of female cohorts arriving in Texas varies from year to year. It should be noted that both nectar and water availability during both the winter and the spring exit from Mexico play a role in survival during these periods.
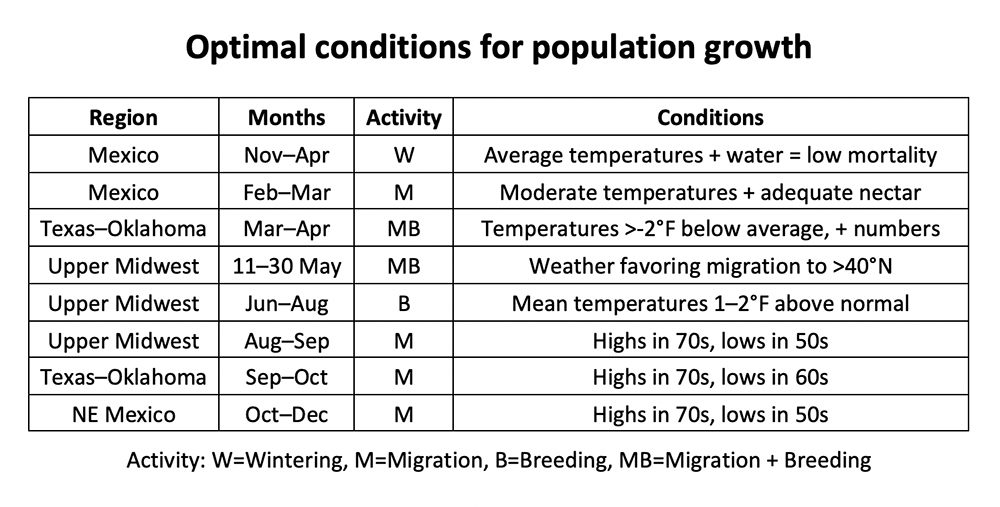
Table 1. Optimal conditions for population growth.
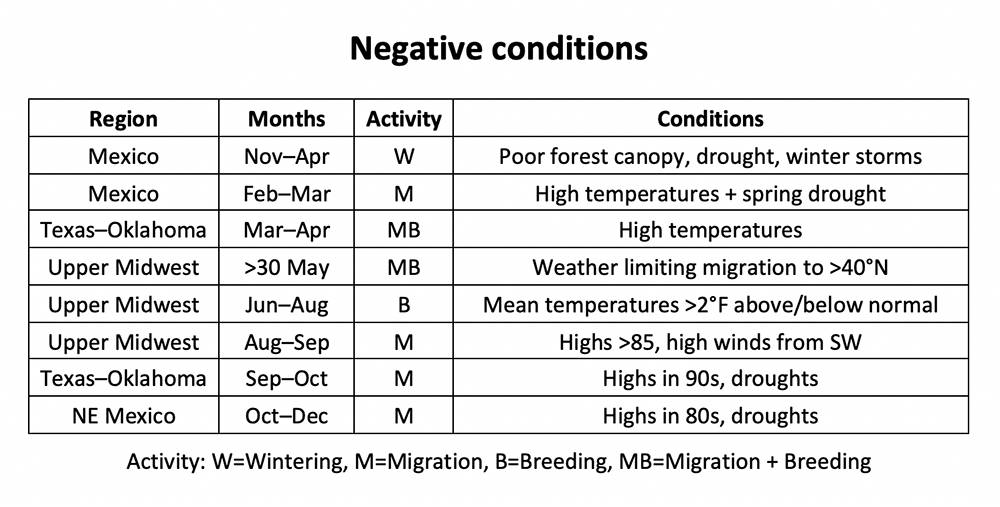
Table 2. Negative conditions.
Once the cohort of returning monarchs reaches the milkweed rich areas of Texas (12-15 March), realized fecundity and age to first reproduction becomes important. Conditions favoring egg laying include temperatures that are less than -1.5°F below the long-term average, abundant emerging milkweeds, adequate nectar and water, moderate winds and relatively low precipitation from 15 March to 15 April. The lower than average temperatures extend the life of the females (lower number of degree days) but also have the effect of limiting northward movement. The net effect of lower temperatures is that most eggs are laid in Texas and southern Oklahoma where it is relatively warm allowing the immatures to grow rapidly – thus reaching reproductive age in a minimum number of days. In contrast, with warmer temperatures females continue moving north laying their eggs at latitudes with cooler temperatures. The effect is to produce offspring with longer ages to first reproduction at these latitudes and to increase the average age to first reproduction for all offspring produced by the cohort arriving from Mexico. The importance of where eggs are laid by returning females and age to first reproduction is supported by the observation that in all four years with mean temperatures of less than -1.5°F for March in Texas, the populations grew from one year to the next. However, the populations declined in 9/11 years during which temperatures were greater than 1.9°F above the mean. One of the two years with high mean March temperatures in which the population increased was 2018 (+5.4°F). In other years with similar high temperatures, the returning monarchs moved northward into Oklahoma, Kansas and sometimes Nebraska, but not in 2018. This unusual dynamic was due to a low that settled over north Texas and southern Oklahoma in late March. Temperatures were low enough during this period to keep monarchs confined to central Texas well into April*. Thus, egg laying was largely confined to Texas where warmer than average temperatures accelerated the development of the immatures. The result was a large cohort of first-generation monarchs that migrated northward in May, a cohort with a low and therefore favorable age to first reproduction. This combination of warm conditions favoring rapid development of immatures yet cold that largely restricted egg laying to Texas in March and into early April has only occurred once since 1994. Yet, it was one of the major factors that contributed to the increase in monarch numbers from 2017 (2.48 hectares) to 2018 (6.05 hectares).
Up to this point I’ve just hinted at how realized fecundity can be modified by extrinsic factors. Let’s consider drought, high temperatures and extended periods of rainfall. There are other factors, but these cases will provide examples of how deviations from optimal conditions can influence population growth.
Summer droughts affect monarchs, nectar sources and host plants. Monarchs need water which is usually obtained from nectar or dew during the summer, and both are scarce in droughts. In addition, monarchs need the carbohydrates (and amino acids) found in nectars to fuel flight, egg development and egg laying, etc. Lack of water and nectar can result in fewer eggs laid and even shorten life span for a reproductive cohort. Higher than average temperatures (>+2°F) can have similar effects. Plants develop faster resulting in shorter flowering intervals, often with lower nectar production and more rapid senescence, the latter making the milkweeds less attractive to females for oviposition. Again, adult life span is reduced at higher temperatures. Rainfall, if prolonged over several days, or any weather that restricts flight and egg laying for a number of days, also reduces realized fecundity. There are only so many degree days in the life of an adult monarch (530)**, time is ticking, and, as pointed out in Zalucki and Rochester (2004), there is no recovery from lost opportunities to lay eggs.
If you have been able to follow this tutorial, it should be apparent how deviations from optimal conditions in terms of realized fecundity are the basis for the stage-specific model I’ve mentioned in previous posts to this Blog. But, it’s not the only factor. We need to consider reproductive success as well. Beyond that, we need to discuss how populations recover from a series of negative conditions that have significantly reduced the size of reproductive cohorts.
*Although the optimal temperatures that allow returning monarchs to move northward are not known, it’s clear that advances are limited when temperatures are less than 70°F.
**The estimated number of degree days (530) represents a life span of roughly 3-4 weeks for reproductive monarchs under average summer conditions (Zalucki and Rochester, 2004). Longer life is possible during periods with daytime temperatures in the 60s. Shorter life spans are expected when temperatures exceed 90°F.
Reference
Zalucki, M.P. and W.A. Rochester. 2004. Spatial and temporal population dynamics of monarchs down under: Lessons for North America. In The Monarch Butterfly: Biology and Conservation, eds., Oberhauser, K. S. and M.J. Solensky. pp. 219-228.
Sorry, comments for this entry are closed at this time.